Potential PhD Topics
PhD Supervisors
Below are listed those CAS staff who may be currently looking for PhD students.
- Dr. Rebecca Allen
- Prof. Matthew Bailes
- Dr. Kirsten Banks
- Prof. Chris Blake
- Prof. Jean Brodie
- A.Prof Michelle Cluver
- Prof. Jeff Cooke
- Prof. Darren Croton
- Dr. Rebecca Davies
- Prof. Adam Deller
- Prof. Alan Duffy
- A.Prof. Deanne Fisher
- Prof. Christopher Fluke
- Dr. Chris Flynn
- Prof. Duncan Forbes
- Prof. Karl Glazebrook
- Prof. Alister Graham
- Prof. Jarrod Hurley
- Prof. Glenn Kacprzak
- Prof. Virginia Kilborn
- A.Prof. Ivo Labbe
- Dr. Marcus Lower
- Dr. Ben McAllister
- Dr. Anais Möller
- Dr. Jade Powell
- Dr. Themiya Nanayakkara
- Prof. Michael Murphy
- Dr. Daniel Reardon
- Prof. Emma Ryan-Weber
- Prof. Ryan Shannon
- Dr. Simon Stevenson
- A.Prof. Edward N. Taylor
- Dr. Sara Webb
PhD Projects
Prof. Matthew Bailes
- No projects offered at this time
Prof. Chris Blake
Prof. Jean Brodie
- No projects offered at this time
A.Prof. Michelle Cluver
- No projects offered at this time
Prof. Jeff Cooke
Prof. Darren Croton
- No projects offered at this time
Dr. Rebecca Davies
- No projects offered at this time
Prof. Adam Deller
Prof. Alan Duffy
- no projects offered at this time
A.Prof. Deanne Fisher
- No projects offered at this time
Dr. Chris Flynn
- No projects offered at this time
Prof. Christropher Fluke
- No projects offered at this time
Prof. Duncan Forbes
- No projects offered at this time
Prof. Karl Glazebrook
Prof. Alister Graham
Prof. Jarrod Hurley
- No projects offered at this time
Prof. Glenn Kacprzak
Prof. Virginia Kilborn
- No projects offered at this time
A.Prof. Ivo Labbe
- No projects offered at this time
Dr. Marcus Lower
- No projects offered at this time
Dr. Ben McAllister
Dr. Anais Möller
- No projects offered at this time
Prof. Michael Murphy
Dr. Themiya Nanayakkara
- No projects offered at this time
Dr. Jade Powell
- No projects offered at this time
Dr Daniel Reardon
Prof. Emma Ryan-Weber
- No projects offered at this time
Prof. Ryan Shannon
Dr. Simon Stevenson
- No projects available at this time
A.Prof. Edward N. Taylor
- No projects offered at this time
Dr. Sara Webb
Project Descriptions
Supervisors: Prof. Chris Blake
The "Lambda CDM" cosmological model, in which the energy of the Universe is dominated by a cosmological constant and cold dark matter, has provided the standard description of the Universe for the past three decades. However, evidence is emerging of discrepancies between different cosmological observations, implying that this model is incomplete. One of the most powerful probes of cosmology is the large-scale structure of the Universe, as mapped by galaxy redshift surveys. This PhD project will use data from the largest such galaxy survey that currently exists, the Dark Energy Spectroscopic Instrument (DESI), to search for clues of new cosmological physics by bridging measurements and theory. Depending on the interests of the student, the project could focus on methods including weak gravitational lensing, galaxy peculiar velocities, and their joint analysis with baryon acoustic oscillations and redshift-space distortions. The project will develop a complete set of research skills including statistical analysis, theory and simulations, and offers collaboration opportunities in one of the current leading international astronomical projects.
Supervisors: Prof. Jeff Cooke
The Swinburne-led Deeper, Wider, Faster (DWF) program is the world’s first all-wavelength program designed to detect and rapidly follow up the fastest transients in the Universe. Fast transients include fast radio bursts, supernova shock breakouts, all types of gamma-ray bursts, kilonovae, flare stars, and many other events, including the potential discovery of unknown classes. DWF is the world's largest collaboration of telescopes, with over 90 major observatories on every continent and in space. For any given operational run, DWF coordinates roughly 10-15 wide-field radio through gamma-ray telescopes to observe the same fields at the same time. Telescopes such as Parkes and ASKAP (radio), CTIO DECam (optical), Astrosat (UV/X-ray), HXMT and Einstein Probe (X-ray), and NASA Neil Gehrels Swift Observatory (UV/X-ray/gamma-ray). These data are processed in real time and transients are identified within minutes of their outbursts in our Swinburne Mission Control room. Our fast transient identification enables rapid-response spectroscopic and imaging follow-up observations before the events fade using the world’s largest telescopes coordinated by the program, such as Keck, the VLT, Gemini-South, SALT, and the AAT (optical), ATCA (radio), and NASA Swift (high-energy), among others. Finally, our network of 1-2 metre-class telescopes located worldwide provide imaging and spectroscopy to monitor the events.
The student will participate in observing runs and analyse the unique multi-wavelength dataset in search of fast transients and early detections of longer-duration events to produce leading science. Depending on the student's interests and experience, the project will involve (1) developing techniques to search the deep, fast-cadenced optical dataset, (2) searching and cross-matching transients in the multi-wavelength datasets, and (3) enhancing transient discovery and analysis by progressing data visualisation and data sonification techniques. Project aims include extending our knowledge of known fast transient types and providing new information on their progenitors, physics, environments, and explosion mechanisms and potentially discovering new fast transient classes.
Supervisors: Prof. Karl Glazebrook
Galaxy kinematics in the high redshift Universe tells us about the evolution of cold dark matter halos and the accumulation of baryons in their centre to make galaxies. These measurements provide for fundamental insights into both cosmology and galaxy evolution models. JWST promises a revolution here thanks to its extreme sensitivity and the high resolution of its images. We are able to measure the motions of stars and gas within galaxies across much greater radial extents and to higher redshifts than were previously possible with the largest ground based telescopes. In this PhD you will learn to work with JWST data from the innovative MSA3D survey, using a ‘slit stepping’ technique we have been able to construct integral field spectroscopic and kinematic maps of ~40 galaxies simultaneously at z~1 and also at z~2. This is the first time galaxy internal motions at these epochs have been probed in such detail. You will use velocity and dispersion maps to measure the angular momentum of galaxies in the high redshift Universe and also their dark matter content and compare with theoretical models. There will also be opportunities to work with the Keck telescopes in Hawaii for complementary observations and propose for new JWST observations. You will be supervised by Prof. Karl Glazebrook (FAA) an internationally recognised leader in the study of high-redshift galaxies who has been working with JWST since its launch, be a member of the international MSA3D team and colocated with the JWST Australian Data Centre team that will provide extensive support for understanding JWST observations.
Figure: JWST and HST image of a z=1.1 spiral galaxy and kinematic maps extracted by the MSA3D team. (Reference: https://doi.org/10.48550/arXiv.2408.08350)
Supervisor: Prof. Alister Graham
There is a largely-missing population of intermediate-mass black holes (IMBHs) with masses higher than that formed by single stars today (Mbh=1.4 to 120 MSun) and less massive than the supermassive black holes (SMBHs: 105—1010 MSun) known to reside at the centres of big galaxies. Not surprisingly, astronomers around the world are hotly pursuing the much-anticipated discovery of IMBHs. This thesis will involve several interconnected projects involving telescope and satellite image analysis and statistical techniques. Improved methods for estimating both IMBH and SMBH masses will be developed and applied, with ties to the upcoming Large Synoptic Survey Telescope expected. The coexistence of these massive black holes in dense, compact star clusters at the centres of galaxies is also expected to be a source gravitational radiation detectable by the planned eLISA satellite, for which updated predictions will be made.
Students will benefit from membership in the ARC Centre of Excellence for Gravitational Wave Discovery, OzGrav.
Supervisors: Prof. Alister Graham
This project will explore how stars are distributed in galaxy images obtained from both ground-based telescopes and satellites such as Hubble and Spitzer. The structure of galaxies reveals much about how they formed, how they are connected with one another and also with the massive black holes that reside in their cores. This knowledge will be used to pursue a number of exciting topics at the forefront of astronomy. A feeling for the type of research done with Prof. Graham can be seen in his Press Releases.
Image: Artistic impression of a black hole featured on the cover of Swinburne University's 2019 annual report. Credit: James Josephides and Alister Graham.
Supervisors: A.Prof. Glenn Kacprzak and Prof. Michael Murphy
Ever wonder why some galaxies form stars while others do not? Or where does all the fuel for star-formation come from and what regulates it? The evolution of galaxies is intimately tied to their gas cycles - the gas accretion, star formation, stellar death and gas expulsion. As galaxies evolve, their gas cycles (known as feedback), give rise to an extended gaseous halo surrounding galaxies. Understanding how feedback works has become recognized as THE critical unknown process missing to fully understand galaxy evolution. Therefore, gaseous galaxy halos are the key astrophysical laboratories harbouring the detailed physics of how galactic feedback governs galaxy evolution. Observationally, galaxy halos are studied with great sensitivity using quasar absorption lines. Imprinted on the quasar spectrum are the motions, chemical content, density, and temperature of the gas. These absorption signatures provide details that are unobtainable using any other method of observation. Here, the student will join an international collaboration and will examine how the host galaxy properties are linked to their circumgalactic gas properties using Hubble Space Telescope and Keck Telescope data.
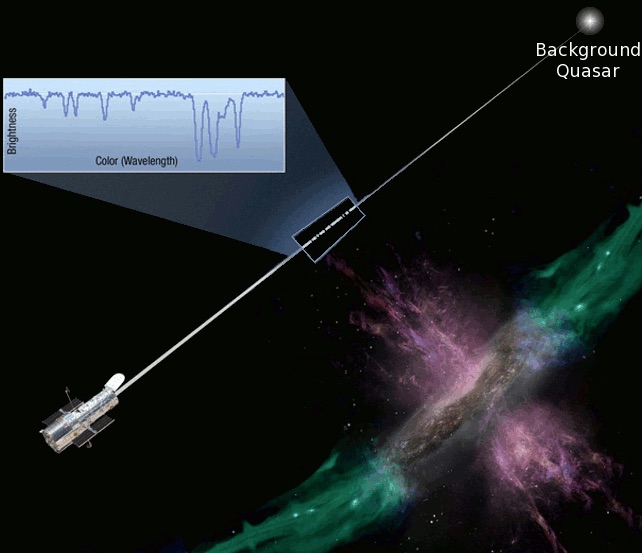
Image Caption: Cool gas (green) from cosmic filaments accretes onto
the galaxy, which drives its rotation and controls the rate at
which it forms stars. Star formation and supernovae expel
gas back into the circumgalatic medium (purple). Background
quasars are used to study these gas flows around galaxies.
Supervisors: Dr. Ben McAllister
The nature of dark matter is one of the biggest mysteries in modern science – it makes up five sixths of the matter in the Universe, and is of unknown composition. It surrounds and passes through the Earth at all times.
Axions are a hypothetical particle, and one of the leading candidates for dark matter. Swinburne is building a new axion detector to try and measure small effects induced by dark matter when it passes through the laboratory, and shed light on the mystery. The kind of experiment we are building is called an axion haloscope.
The detector is currently being constructed and will be hosted at Swinburne. There is work to be done on various aspects of the project, from optimal detector design, to manufacturing and characterisation, to advanced readout technology, to control software and data analysis.
This project could focus on any of these areas, tailored to fit the skills and interests of the candidate. There is room for multiple students, and you will be working in a small team with other researchers. For example, this project could include aspects of mechanical and RF design, material science, computational modelling, software to control the detector and associated equipment, or on a pipeline to acquire and tease through experimental data for hints of new physics.
Supervisors: Prof. Ryan Shannon, Prof. Adam Deller, Dr. Daniel Reardon, and Dr. Matt Miles (Vanderbilt)
Pulsar timing arrays are Galactic-scale gravitational wave detectors that detect and study gravitational wave emission from the most massive black holes in the Universe: supermassive black holes in binaries in the throes of merger, embedded in the centres of galaxies. Major pulsar timing array experiments recently announced compelling evidence for a gravitational-wave background: the sum of gravitational waves from all of the binary supermassive black holes in the Universe. This discovery has demonstrated the power of PTAs as a new tool to study the Universe. After a background is detected, the next signal to seek is that from an individual supermassive black hole binary.
In this project, you will develop algorithms and undertake searches for individual binary supermassive black holes with state of the art pulsar timing array data sets, including that from the Swinburne-led (MeerKAT pulsar timing array project. You will first develop methods to optimise the sensitivity of pulsars timing array to individual binary supermassive black holes. This will include using precise pulsar distance measurements to improve searches for individual black hole binaries, and sophisticated modelling to correct for systematics introduced by the interstellar medium. You will then conduct searches for individual sources with the MeerKAT PTA and International Pulsar Timing Array data sets. The results of the searches will be compared to models to better understand the demographics of supermassive black holes and how they shape galaxy formation and evolution.
This project is part of the ARC Centre for Excellence for Gravitational Wave Discovery (OzGrav): a virtual research centre that brings together researchers and students in Australian and around the world to undertake cutting edge research in gravitational waves and fundamental physics and astrophysics.
Image Credit: Carl Knox (Swinburne/OzGrav)
Supervisors: Dr. Sara Webb
The night sky is a dynamic and changing environment, with new and evolving sources known as transients, detected each night. Transients can be identified in large optical surveys due to their changing brightness over time. The most well-known and studied transients are astrophysical in nature, caused by bursts of radiation emitted by exploding or flaring stars and other high energy astrophysical events. However, there is a population of Short Duration Optical Transients (SDOTs), lasting for only seconds to minutes, which are currently poorly understood. These SDOTs are often only detected in single telescope image exposures and are notoriously challenging to follow-up and constrain their origins and properties. Often SDOT events with only single detections are discarded or filtered out in our transient search algorithms for large optical astronomical surveys. It is vital for us to prevent SDOTs being overlooked in upcoming all-sky surveys and uncover their true origins.
The HDR candidate will work with Dr. Sara Webb to uncover the mystery of SDOTs using the largest optical surveys in the world. In 2007 the first millisecond radio transient was discovered and dubbed a Fast Radio Bursts (FRB) (Lorimer et al, 2007). Currently, 1000 (published) FRB events have been detected, with only a fraction of these events observed to repeat (i.e; Spilter et al, 2017, Kumar et al., 2019, Fonseca et al., 2020). The properties of these events have been studied in-depth, aiming to determine the likely origin of the bursts. The Dispersion Measure (DM) of the FRB pulse is used to probe the sources estimated distance (Cordes et al., 2019). It became apparent that the vast majority of FRBs had high DMs suggesting extragalactic origins. Although there exist multiple progenitor theories, no definitive mechanism has been defined (Platts et al., 2019). To fully probe FRBs and their progenitors, a detailed understanding of SDOTs is essential. This is where the Vera C. Rubin Observatory will become curial to understand statistical rates and correlation with FRB rates across the sky, and rejection of artificial events caused by space debris glints.
The HDR candidate will work with data from multiple optical surveys, propose for follow-up telescope time and process data on Swinburne's Supercomputing facilities. In this project you can expect to data mine rich optical datasets for SDOT candidates, produce machine learning algorithms for classification of SDOTs and work alongside expert astronomers across multiple wavelength regimes to investigate these phenomena.