Potential PhD Topics
PhD Supervisors
Below are listed those CAS staff who may be currently looking for PhD students.
- Dr. Rebecca Allen
- Prof. Matthew Bailes
- Dr. Kirsten Banks
- Prof. Chris Blake
- Prof. Jean Brodie
- A.Prof Michelle Cluver
- Prof. Jeff Cooke
- Prof. Darren Croton
- Dr. Rebecca Davies
- Prof. Adam Deller
- Prof. Alan Duffy
- A.Prof. Deanne Fisher
- Prof. Christopher Fluke
- Dr. Chris Flynn
- Prof. Duncan Forbes
- Prof. Karl Glazebrook
- Prof. Alister Graham
- Prof. Jarrod Hurley
- Prof. Glenn Kacprzak
- Prof. Virginia Kilborn
- A.Prof. Ivo Labbe
- Dr. Marcus Lower
- Dr. Ben McAllister
- Dr. Anais Möller
- Dr. Jade Powell
- Dr. Themiya Nanayakkara
- Prof. Michael Murphy
- Dr. Daniel Reardon
- Prof. Emma Ryan-Weber
- Prof. Ryan Shannon
- Dr. Simon Stevenson
- A.Prof. Edward N. Taylor
- Dr. Sara Webb
PhD Projects
Prof. Matthew Bailes
- No projects offered at this time
Prof. Chris Blake
Prof. Jean Brodie
- No projects offered at this time
A.Prof. Michelle Cluver
- No projects offered at this time
Prof. Jeff Cooke
- The Deeper, Wider, Faster program: Discovering the fastest bursts in the Universe
- Discovering the most extreme explosions to probe the early Universe
Prof. Darren Croton
- No projects offered at this time
Dr. Rebecca Davies
Prof. Adam Deller
- No projects offered at this time
Prof. Alan Duffy
- no projects offered at this time
A.Prof. Deanne Fisher
Dr. Chris Flynn
- No projects offered at this time
Prof. Christropher Fluke
- No projects offered at this time
Prof. Duncan Forbes
Prof. Karl Glazebrook
Prof. Alister Graham
Prof. Jarrod Hurley
- No projects offered at this time
Prof. Glenn Kacprzak
Prof. Virginia Kilborn
- No projects offered at this time
A.Prof. Ivo Labbe
- No projects offered at this time
Dr. Marcus Lower
- No projects offered at this time
Dr. Ben McAllister
Dr. Anais Möller
- No projects offered at this time
Prof. Michael Murphy
- Do the fundamental constants of nature actually vary?
- Understanding gas flows in and around galaxies
Dr. Themiya Nanayakkara
- No projects offered at this time
Dr. Jade Powell
- No projects offered at this time
Dr Daniel Reardon
- No projects offered at this time
Prof. Emma Ryan-Weber
- No projects offered at this time
Prof. Ryan Shannon
- No projects offered at this time
Dr. Simon Stevenson
- No projects available at this time
A.Prof. Edward N. Taylor
Dr. Sara Webb
- No projects offered at this time
Project Descriptions
Supervisors: Prof. Chris Blake
The "Lambda CDM" cosmological model, in which the energy of the Universe is dominated by a cosmological constant and cold dark matter, has provided the standard description of the Universe for the past three decades. However, evidence is emerging of discrepancies between different cosmological observations, implying that this model is incomplete. One of the most powerful probes of cosmology is the large-scale structure of the Universe, as mapped by galaxy redshift surveys. This PhD project will use data from the largest such galaxy survey that currently exists, the Dark Energy Spectroscopic Instrument (DESI), to search for clues of new cosmological physics by bridging measurements and theory. Depending on the interests of the student, the project could focus on methods including weak gravitational lensing, galaxy peculiar velocities, and their joint analysis with baryon acoustic oscillations and redshift-space distortions. The project will develop a complete set of research skills including statistical analysis, theory and simulations, and offers collaboration opportunities in one of the current leading international astronomical projects.
Supervisors: Prof. Jeff Cooke
The Swinburne-led Deeper, Wider, Faster (DWF) program is the world’s first all-wavelength program designed to detect and rapidly follow up the fastest transients in the Universe. Fast transients include fast radio bursts, supernova shock breakouts, all types of gamma-ray bursts, kilonovae, flare stars, and many other events, including the potential discovery of unknown classes. DWF is the world's largest collaboration of telescopes, with over 90 major observatories on every continent and in space. For any given operational run, DWF coordinates roughly 10-15 wide-field radio through gamma-ray telescopes to observe the same fields at the same time. Telescopes such as Parkes and ASKAP (radio), CTIO DECam (optical), Astrosat (UV/X-ray), HXMT and Einstein Probe (X-ray), and NASA Neil Gehrels Swift Observatory (UV/X-ray/gamma-ray). These data are processed in real time and transients are identified within minutes of their outbursts in our Swinburne Mission Control room. Our fast transient identification enables rapid-response spectroscopic and imaging follow-up observations before the events fade using the world’s largest telescopes coordinated by the program, such as Keck, the VLT, Gemini-South, SALT, and the AAT (optical), ATCA (radio), and NASA Swift (high-energy), among others. Finally, our network of 1-2 metre-class telescopes located worldwide provide imaging and spectroscopy to monitor the events.
The student will participate in observing runs and analyse the unique multi-wavelength dataset in search of fast transients and early detections of longer-duration events to produce leading science. Depending on the student's interests and experience, the project will involve (1) developing techniques to search the deep, fast-cadenced optical dataset, (2) searching and cross-matching transients in the multi-wavelength datasets, and (3) enhancing transient discovery and analysis by progressing data visualisation and data sonification techniques. Project aims include extending our knowledge of known fast transient types and providing new information on their progenitors, physics, environments, and explosion mechanisms and potentially discovering new fast transient classes.
Supervisors: Prof. Jeff Cooke
Very massive stars are predicted to end their lives as extremely bright explosions called core-collapse supernovae. These events are very bright and, with current technology, can be observed well into the distant Universe, corresponding to a lookback time when it was only about 10% its current age. Superluminous supernovae are a recently discovered class that are 10-100 times more luminous than core-collapse supernovae and can be observed back to a time when the Universe was about 1-2% its current age. Superluminous supernovae are brighter than their host galaxies and are a means to detect the deaths of the very first generation of stars (the Population III stars) to have formed shortly after the Big Bang and possibly the elusive pair-instability supernovae, a third type of supernova predicted back in the 1960s but are expected to occur in the early Universe. Core-collapse and superluminous supernovae are powerful tools to understand the formation rates of stars, neutron stars, and black holes in the early Universe, they impact galaxy formation and the reionisation of the Universe, they provide one or our only means to probe the ‘invisible’ gas between galaxies in the early Universe, and they show promise as tools to measure the expansion rate of the Universe across all cosmic time.
In this project, the student will use existing, and acquire new, data from the Subaru, Keck, and ESO observatories and the NASA Hubble and James Webb and soon-to-be-launched Roman Space Telescopes to discover and study core-collapse and superluminous supernovae in the early Universe. Main scientific aims will be to constrain what types of stars create them, the properties of their host galaxies, the conditions in the early Universe, and to help establish a far-ultraviolet supernova classification scheme that will be used by all distant supernova discoveries in the future.
Image caption: Artist's animation of a superluminous supernova and possibly a pair-instability supernova. Credit: M. Weiss. Right: Artist's conception of a supernova explosion in a galaxy in the early Universe. Credit: A. Malec, M. Martig (Swinburne).
Supervisors: Dr. Rebecca Davies
Galactic outflows are violent ejections of gas from galaxies triggered by exploding stars. Outflows have enormous impacts on the galaxies they come from. They remove large amounts of hydrogen gas, depriving galaxies of fuel to form new stars. Most galaxy evolution theories predict that this removal of gas plays a fundamental role in regulating galaxy properties. Outflows also transport elements like carbon and oxygen between galaxies and across intergalactic space, sending life-critical elements throughout the Universe. Outflows are difficult to study: they are much fainter than galaxies (see upper picture), so many key questions about their properties and their impact on galaxy evolution remain unanswered. This project will use new state-of-the-art observations from facilities such as the James Webb Space Telescope, the WM Keck Telescopes and ESO’s Very Large Telescope to measure the properties of galactic outflows in the early Universe and explore whether they could be responsible for creating massive quiescent gaaxies. The student will join a vibrant research team at Swinburne and will have opportunities to assist with Keck observations as well as travel overseas for conferences and visits to international collaborators.
Supervisors: Prof. Duncan Forbes
In 2015 a new class of galaxy was discovered - the ghostly Ultra Diffuse Galaxy (UDG). Such galaxies have the same total luminosity as a dwarf galaxy but some reveal a halo of dark matter similar to that of a giant galaxy making them 99% dark matter! Since their discovery they remain a mystery and a challenge to theories of galaxy formation. This PhD project aims to discover more UDGs from deep imaging, determine their stellar population and dynamical properties, and compare them with the latest theoretical models. This project is an observational one, using new data from the world's largest telescopes (Keck and the VLTs) and involving colleagues in California.
Image caption: Ultra Diffuse Galaxies have the same total luminosity as a dwarf galaxy but the same total mass as a giant galaxy. Some UDGs are 99% composed of dark matter and we don't know why! (Credit: Schoening/Harvey/van Dokkum/NASA/ESA Hubble Space Telescope.
Supervisors: Prof. Karl Glazebrook
Galaxy kinematics in the high redshift Universe tells us about the evolution of cold dark matter halos and the accumulation of baryons in their centre to make galaxies. These measurements provide for fundamental insights into both cosmology and galaxy evolution models. JWST promises a revolution here thanks to its extreme sensitivity and the high resolution of its images. We are able to measure the motions of stars and gas within galaxies across much greater radial extents and to higher redshifts than were previously possible with the largest ground based telescopes. In this PhD you will learn to work with JWST data from the innovative MSA3D survey, using a ‘slit stepping’ technique we have been able to construct integral field spectroscopic and kinematic maps of ~40 galaxies simultaneously at z~1 and also at z~2. This is the first time galaxy internal motions at these epochs have been probed in such detail. You will use velocity and dispersion maps to measure the angular momentum of galaxies in the high redshift Universe and also their dark matter content and compare with theoretical models. There will also be opportunities to work with the Keck telescopes in Hawaii for complementary observations and propose for new JWST observations. You will be supervised by Prof. Karl Glazebrook (FAA) an internationally recognised leader in the study of high-redshift galaxies who has been working with JWST since its launch, be a member of the international MSA3D team and colocated with the JWST Australian Data Centre team that will provide extensive support for understanding JWST observations.
Figure: JWST and HST image of a z=1.1 spiral galaxy and kinematic maps extracted by the MSA3D team. (Reference: https://doi.org/10.48550/arXiv.2408.08350)
Supervisor: Prof. Alister Graham
There is a largely-missing population of intermediate-mass black holes (IMBHs) with masses higher than that formed by single stars today (Mbh=1.4 to 120 MSun) and less massive than the supermassive black holes (SMBHs: 105—1010 MSun) known to reside at the centres of big galaxies. Not surprisingly, astronomers around the world are hotly pursuing the much-anticipated discovery of IMBHs. This thesis will involve several interconnected projects involving telescope and satellite image analysis and statistical techniques. Improved methods for estimating both IMBH and SMBH masses will be developed and applied, with ties to the upcoming Large Synoptic Survey Telescope expected. The coexistence of these massive black holes in dense, compact star clusters at the centres of galaxies is also expected to be a source gravitational radiation detectable by the planned eLISA satellite, for which updated predictions will be made.
Students will benefit from membership in the ARC Centre of Excellence for Gravitational Wave Discovery, OzGrav.
Supervisors: Prof. Alister Graham
This project will explore how stars are distributed in galaxy images obtained from both ground-based telescopes and satellites such as Hubble and Spitzer. The structure of galaxies reveals much about how they formed, how they are connected with one another and also with the massive black holes that reside in their cores. This knowledge will be used to pursue a number of exciting topics at the forefront of astronomy. A feeling for the type of research done with Prof. Graham can be seen in his Press Releases.
Image: Artistic impression of a black hole featured on the cover of Swinburne University's 2019 annual report. Credit: James Josephides and Alister Graham.
Supervisors: A.Prof. Deanne Fisher and Prof. Glenn Kacprzak
In starburst galaxies, supernovae explosions push gas up out of the galaxy and into the cosmos above. We call these `galactic winds’. Galactic winds change the properties of the galaxy, and are considered by most theories to be a linchpin that regulates the growth of galaxies. Yet, there is not currently any theory that successfully explains their properties. This is, therefore, a very active field of research that will place you well for future work. Our team views this as faint filaments of gas that extends above star forming galaxies using extremely deep observations on the world's largest telescopes available. The physical properties of the gas directly relate to the physical models of how these large outflows of gas evolve and shape outflows. We will use data from the Keck 10 meter telescope, the VLT 8 meter telescope and the ALMA radio telescope to study these exploding galaxies. You will work in a team of other PhD students and postdocs who work within our group. We will both use data that is ready for you to process, and make new observations on these large telescopes.
Further information:
- Galactic winds dictating galaxy evolution: a 20 min Lecture by L. Zscaechner
- How feedback shapes galaxy evolution: a 1 hour lecture by Prof Christy Tremonti
Supervisors: A.Prof. Glenn Kacprzak and Prof. Michael Murphy
Ever wonder why some galaxies form stars while others do not? Or where does all the fuel for star-formation come from and what regulates it? The evolution of galaxies is intimately tied to their gas cycles - the gas accretion, star formation, stellar death and gas expulsion. As galaxies evolve, their gas cycles (known as feedback), give rise to an extended gaseous halo surrounding galaxies. Understanding how feedback works has become recognized as THE critical unknown process missing to fully understand galaxy evolution. Therefore, gaseous galaxy halos are the key astrophysical laboratories harbouring the detailed physics of how galactic feedback governs galaxy evolution. Observationally, galaxy halos are studied with great sensitivity using quasar absorption lines. Imprinted on the quasar spectrum are the motions, chemical content, density, and temperature of the gas. These absorption signatures provide details that are unobtainable using any other method of observation. Here, the student will join an international collaboration and will examine how the host galaxy properties are linked to their circumgalactic gas properties using Hubble Space Telescope and Keck Telescope data.
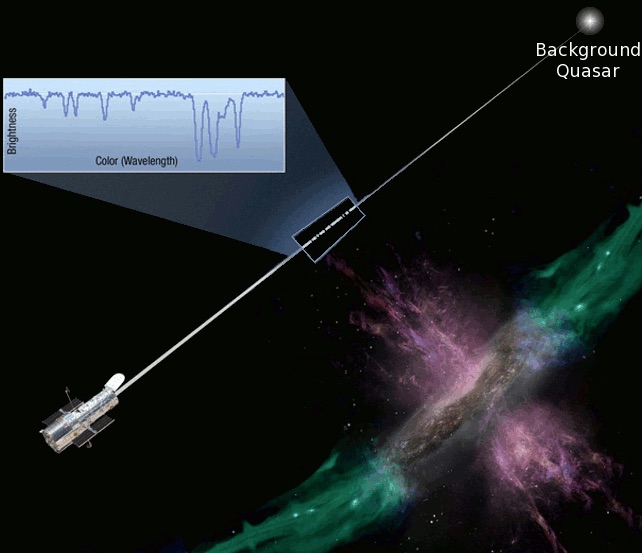
Image Caption: Cool gas (green) from cosmic filaments accretes onto
the galaxy, which drives its rotation and controls the rate at
which it forms stars. Star formation and supernovae expel
gas back into the circumgalatic medium (purple). Background
quasars are used to study these gas flows around galaxies.
Supervisors: Dr. Ben McAllister
The nature of dark matter is one of the biggest mysteries in modern science – it makes up five sixths of the matter in the Universe, and is of unknown composition. It surrounds and passes through the Earth at all times.
Axions are a hypothetical particle, and one of the leading candidates for dark matter. Swinburne is building a new axion detector to try and measure small effects induced by dark matter when it passes through the laboratory, and shed light on the mystery. The kind of experiment we are building is called an axion haloscope.
The detector is currently being constructed and will be hosted at Swinburne. There is work to be done on various aspects of the project, from optimal detector design, to manufacturing and characterisation, to advanced readout technology, to control software and data analysis.
This project could focus on any of these areas, tailored to fit the skills and interests of the candidate. There is room for multiple students, and you will be working in a small team with other researchers. For example, this project could include aspects of mechanical and RF design, material science, computational modelling, software to control the detector and associated equipment, or on a pipeline to acquire and tease through experimental data for hints of new physics.
Supervisors: Prof. Michael Murphy
The fundamental constants characterise the strength of nature's forces. Their constancy has been tested in laboratories to extraordinary precision, and they have been mapped across the universe. But new theories, that go beyond the standard model of particle physics, suggest these "constants" may vary where dark matter is highly concentrated, like the centres of galaxies, including our own. This PhD project will be part of an ongoing effort to map the fine-structure constant – alpha, the strength of electromagnetism – closer to our Milky Way's Centre. To measure alpha, we carefully compare spectra of stars near the Centre, about 23,000 light-years away, with very similar stars in our local region. We are currently observing these stars with the Keck Observatory (Hawai`i), of which Swinburne is a partner, and the Very Large Telescope (Chile). Depending on the interests of the student, there can be observational or theoretical avenues to explore in this PhD project: the student may participate in the astronomical observations, data analysis and measurement of alpha, or they could work on advanced quantum mechanical calculations to determine how the spectra of stars depend on fundamental constants. These and other options will be discussed with the candidate. Further reading: Murphy et al. (2022, Science, 378, 634)
Supervisors: A.Prof. Edward N. Taylor
The 4MOST Hemisphere Survey (4HS) will measure optical spectra and redshifts for 4.5 million galaxies across the entire southern hemisphere, with particular emphasis on galaxies in the relatively nearby (z < 0.15) Universe. The primary cosmology goal for 4HS is to map not only the cosmic density field – i.e. where galaxies are – but also the cosmic velocity field: real motions of galaxies through space, above and beyond the Hubble flow. The velocity field provides an instantaneous snapshot of the gravitational collapse of large-scale structure and so measures the cosmological growth rate of structure, which is an essential cosmological parameter (like H0 or Omega-matter). Even better: with a map of both the density field and also the velocity field, it becomes possible to measure gravity over the largest possible scales (from millions to billions of lightyears). Within its first year of operations (i.e. by the end of 2026), 4HS will already be the best PV cosmology dataset ever assembled, in terms of number, volume, and quality; at the end of the 5-year survey period, when combined with other existing datasets, 4HS will complete the map of the density and velocity fields of the local Universe.
In this project, you will have the chance to develop and apply cutting-edge statistical tools to derive the distance and velocity estimates that are the cornerstone of the 4HS cosmology project. The key to the project is an ingenious and super-efficient strategy for fitting and inference that is called the NUTS, which is the new standard for frontline data science packages like numpyro or pyMC. As well as learning powerful and transferable data skills, you will have the chance to make real and high-profile contributions within our global (and super friendly!) 4HS cosmology science working group, and especially with Australian collaborators including Matthew Colless (ANU) and Cullan Howlett and Khaled Said(UQ). This project has guaranteed funding (including travel) through an ARC Discovery Project.